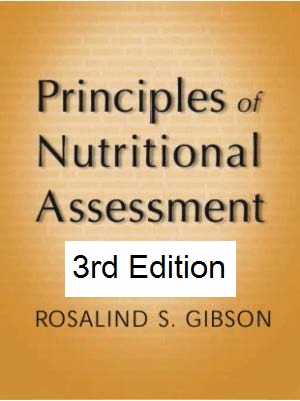
de Groot L.G.P.G.M. and
Gibson R.S. Principles of
Nutritional Assessment:
Vitamin B12
3rd Edition. November, 2024
Abstract
Dietary deficiencies of vitamin B12 in industrialized countries are relatively rare and usually arise from food-bound malabsorption, particularly in the elderly. Secondary B12 deficiency may also develop from certain disease states or medications that interfere with B12 absorption. In low or middle-income countries, however, dietary vitamin B12 deficiency may occur among children and pregnant and lactating women who exclude or consume low amounts of animal-source foods. Absorption of B12 occurs via passive or active absorption, the latter a complex process during which the bioavailability of dietary B12 in healthy adults is assumed to be about 40‑50%. Dietary requirements for B12 vary across countries, with some establishing adequate intakes (AIs) but not requirements per se. There is no Tolerable Upper Intake Level (UL) for vitamin B12 because high intakes have not been associated with any adverse health effects. There are two major metabolic roles for vitamin B12: (a) synthesis of methionine from homocysteine and (b) conversion of methylmalonyl coenzyme A to succinyl coenzyme A. Vitamin B12 has an important role in cellular metabolism, especially in DNA-synthesis, methylation and mitochondrial metabolism. Deficiency of B12 predominantly affects hematological and neurological systems, although neuropsychiatric symptoms are often the first clinical manifestation. Notably, the full spectrum of clinical features associated with B12 deficiency is much broader, is nonspecific, and may occur as a consequence of other diseases. Risk of B12 insufficiency can be assessed through measurement of dietary vitamin B12 intake, a biomarker of exposure. Currently, no gold standard exists to define deficiency of vitamin B12. Total B12 concentration in serum is most frequently used, despite its poor sensitivity and specificity. Consequently, a combination of biomarkers is preferred to define and classify B12 status. If subclinical vitamin B12 is suspected, then circulating total vitamin B12 or transcobalamin (TC)-bound B12 (i.e., holo TC) in serum should be measured first, followed by methylmalonic acid (MMA) or homocysteine in serum, both more sensitive functional biomarkers that reflect B12 stores rather than intake. Algorithms based on all or some of these biomarkers measured either sequentially or concurrently are often applied, with concurrent biomarkers combined into a single indicator termed cB12. For all B12 biomarkers, information should be collected on age, gender, ethnicity, pregnancy, folate status, and presence of possible bacterial overgrowth because all these factors impact B12 biomarkers. There is no consensus on the interpretive criteria used to define inadequate B12 status with these B12 biomarkers. For some, statistically defined reference limits are used, whereas for others "true" cutoffs" based on a known relationship between the B12 biomarker and low body stores, functional impairment, or clinical signs of deficiency, are applied. Often in clinical patients, once B12 deficiency is diagnosed, the next step is to establish whether malabsorption is the cause. In the past, the Schilling test was used, but is rarely conducted today because this test uses radioactive B12. Instead, the CobaSorb test that measures change in serum holoTC concentrations following an oral dose of unlabeled B12 can be used. Under development is the 14C or 13C-labeled absorption test, each of which measures enrichment of 14C or 13C, respectively in blood, urine, or stool following oral ingestion of microbiologically-produced B12 labeled with 14Carbon or 13Carbon. CITE AS: de Groot L.G.P.G.M. & Gibson R.S., Principles of Nutritional Assessment: Vitamin B12.https://nutritionalassessment.org/b12/
Email: Rosalind.Gibson@Otago.AC.NZ
Licensed under CC-BY-4.0
( PDF )
22b.1 Introduction
The term "vitamin B12" is a generic descriptor for all the corrinoids (compounds containing the corrin nucleus) that exhibit the biological activity of cyano-cobalamin. The latter was first isolated in the crystalline form in 1948 (Rickes et al., 1948; Smith, 1948) and the structure, described by Hodgkin et al. (1956), is shown in Figure 22b.1. Here, a cyano-group is linked to a cobalt nucleus. Different forms of cobalamin exist with linkages of methyl, adenosyl or hydroxo groups to cobalt, resulting in methylcobalamin, adenosylcobalamin and hydroxocobalamin, respectively.
22b.1.1 Functions of vitamin B12
There are two naturally occurring cobalamin containing co-enzymes: methylcobalamin, the main form in plasma, and 5‑deoxyadenosylcobalamin, found in the liver, most body tissues, and foods. In humans, methylcobalamin functions in the folate-dependent methylation of homocysteine to methionine (Figure 22b.2) whereas 5'‑deoxyadenosyl-cobalamin plays a major part in the conversion of L‑methylmalonyl-coenzyme A to succinyl-coenzyme A in an isomerization reaction. The latter is a common pathway for the degradation of certain amino acids and odd-chain fatty acids. Vitamin B12 also has a link with nucleic acid metabolism via its role in the conversion of 5‑methyltetrahydrofolate to tetrahydrofolate. Consequently, a deficiency of vitamin B12, like folate, impairs the production of tetrahydrofolate, which is required for thymidine and thus DNA synthesis. Impaired DNA synthesis is responsible for the megaloblastic bone marrow, a characteristic of deficiencies of both vitamin B12 and folic acid. Figure 22b.2.
22b.1.2 Food sources and dietary intakes
In nature, vitamin B12 is produced almost entirely by bacterial synthesis. Vitamin B12-producing bacteria colonize the large bowel, but humans do not have the ability to absorb bacterial vitamin B12 from their large intestine. Instead, humans are dependent solely on dietary sources of vitamin B12 from: (a) methyl- and 5'deoxyadenosyl forms of vitamin B12 present in animal-source foods, which reach the small intestine (Sobczynska-Malefora & Smith, 2022) or (b) crystalline B12 in fortified foods or dietary supplements. Plants do not contain vitamin B12, except for some dried green and purple seaweeds, which have a symbiotic relation with B12 producing bacteria (Allen et al., 2018). The richest food sources of vitamin B12 include meat (especially liver, followed by kidney and heart), eggs, dairy products, fish and shellfish. Several forms of vitamin B12 exist in foods: meat and fish contain mainly adenosyl-cobalamin (Ado‑Cbl) and hydroxocobalamin (OH‑Cbl). Organo-cobalamin (OG‑Cbl) is found mostly in milk; and methyl-cobalamin (Me‑Cbl), Ado‑Cbl, and OH‑Cbl are found in nearly all dairy products (Sobczynska-Malefora et al., 2021). Vitamin B12 is fairly stable and normally not destroyed by cooking at normal temperatures, although in an alkaline pH some loss may occur. Bioavailability of vitamin B12 from foods is generally assumed to be 40‑50% for healthy adults. However, studies assessing bioavailability of B12 from specific food sources in healthy participants have shown that absorption varies depending on both the specific food product and the magnitude of the dose, ranging from 36%‑24% for egg products (dose 0.3‑0.94mg, respectively), 83%‑52% for lean meat (dose 0.54‑5.11mg, respectively), 42%‑30% for fish (dose 2.1‑13.1mg, respectively) and 49%‑4.5% for liver products (dose 0.5‑38mg, respectively) (Doets et al., 2013). Early approaches for studying the bioavailability of B12 involved radio-actively labeled B12. New methods under development for studying the active absorption of B12 from different foods include the use of 14C‑labeled B12 (Brito et al., 2018) and the stable isotope 13C‑labeled B12 (Devi et al., 2020). The uptake of supplemental (crystalline) vitamin B12 from B12‑fortifed foods and dietary supplements occurs by passive diffusion.22b.1.3 Absorption and metabolism
For the transport into body cells, vitamin B12 is absorbed by either active absorption or passive diffusion. Only 1‑2% of an oral crystalline dose of non-protein bound vitamin B12 — as occurs in fortified foods and supplements — is absorbed by simple passive diffusion throughout the length of the gastrointestinal tract (Doets et al., 2013). Active absorption of vitamin B12 is a highly complex active transport system, which takes 3‑4h to complete from ingestion by mouth to appearance in the circulation. Essential steps include the release of dietary B12 from the food matrix by the activity of hydrochloric acid and gastric protease in the stomach. Here B12 binds to haptocorrin produced by salivary glands. In the upper small intestine where the pH changes to less acidic conditions, haptocorrin is degraded by pancreatic proteases, releasing B12 which subsequently becomes bound to gastric intrinsic factor (IF) to form the IF‑B12 complex. Gastric intrinsic factor is a highly specific binding glycoprotein, which is synthesized and secreted by the parietal cells of the gastric mucosa. Absorption of the IF‑B12 complex mainly takes place through receptor sites in the terminal ileum, at an alkaline pH, and in the presence of calcium ions (Allen et al., 2018; Herbert, 1987). However, the capacity of IF‑mediated active absorption is rate limited, as the IF‑B12 ileal receptors become saturated at B12 intakes of about 1.5‑2.5µg from a single meal (Scott, 1997). Reports of isotope studies, including the use of the stable isotope 13C‑labeled B12 (Devi et al., 2020), indicate that 50% of an oral dose of 1µg is absorbed, 20% of a 5µg dose, and 5% of a 25µg dose, as shown in Figure 22b.3.
22b.1.4 Deficiency of vitamin B12 in humans
The common consequence of B12 deficiency is a cellular deficit in one of the coenzyme forms of B12 which affects cellular metabolism, methylation and mitochondrial metabolism: such deficits may pass from a stage of subclinical deficiency through to severe, clinical forms. The clinical signs of vitamin B12 deficiency occur in the hematopoietic, gastrointestinal, and neurological systems Figure 22b.4.
22b.1.5 Recommended intakes of vitamin B12
When setting recommended dietary reference values, expert groups assume the bioavailability of B12 from the diet is about 50% for healthy adults with normal absorption, even though the absorption from specific foods varies markedly as noted earlier. Absorption of crystalline B12 from fortified foods and dietary supplements is higher ranging from 55 to 74%, and unaffected by food-bound malabsorption. Currently there is some debate about whether the current B12 recommendations are sufficient to achieve optimum vitamin B12 status (Doets et al., 2013). Differences exist across expert groups on both the approaches used, and the recommended B12 levels set. For a summary table comparing the recommendations across countries, see Allen et al. (2018). In the United States and Canada, Dietary Reference Intakes (DRIs) were set to maintain hematological values of serum vitamin B12 within the normal range, whereas the European Food Safety Authority (EFSA) concluded there was insufficient available data to set DRIs (EFSA, 2015). Instead, EFSA used a combination of B12 biomarkers and European adult mean B12 intakes to estimate Adequate Intakes (AIs) for all life-stage and physiological groups. In the United States and Canada, the Estimated Average Requirement (EAR) for both males and females aged 19 >70y is 2µg/day. The EAR represents the level of vitamin B12 that is estimated to meet the B12 requirements of 50% of the healthy individuals in a particular sex and life-stage group. The corresponding Recommended Dietary Allowance (RDA) for these adults is 2.4µg/day (assuming a CV of 10%), a level designed to cover the needs of 97 to 98 percent of individuals in the group. However, because of the high prevalence of food-bound malabsorption among older adults, those >50 years are encouraged to consume B12 fortified foods (such as fortified ready-to-eat cereals) or supplements to ensure they meet their B12 needs. For details of the DRIs for other life-stage groups, see IOM (2000) and Allen et al. (2018). WHO/FAO (2004) has adopted the DRIs for B12 set by IOM (2000). In contrast, the AIs set by EFSA (2015) as an intake goal for individuals in all life-stage and physiological groups, are higher than those set by the IOM (2000). and WHO/FAO (2004) and depending on age, range from 1.5 and 4.0µg/day, for adults. For pregnant and lactating women, the AIs are 4.5 and 5.0µg/day, respectively. The AIs for infants and children were calculated by extrapolation from the AI for adults. For more information on the B12 intakes from nine European countries, see EFSA (2015). For more details on the correct use of these differing dietary B12 recommendations, see Chapter 8b: Evaluation of nutrient intakes and diets.22b.1.6 Effects of high intakes of vitamin B12
There appears to be no evidence that excessive intakes of vitamin B12 from food or supplements causes adverse effects in healthy persons (IOM, 2000). Consequently, both the U.S. Food and Nutrition Board (IOM, 2000) and EFSA (2024). have concluded there are insufficient data to set a Tolerable Upper Intake Level (UL) for vitamin B12.22b.2 Biomarkers of vitamin B12 status
Biomarkers of vitamin B12 status have been reviewed by the expert international panel as part of the Biomarkers of Nutrition for Development (BOND) project; see Allen et al (2018) for more details. Risk of B12 insufficiency can be assessed through the measurement of dietary intake of vitamin B12, a biomarker of exposure. Quantitative dietary assessment methods such as 24h recalls and food records must be used; for more details see Chapter 3: Measurement of food consumption of individuals. The variation in day-to-day intake of vitamin B12 is often large when vitamin B12‑rich foods such as liver are consumed, so multiple days of intake should be recorded on each individual and the observed distribution of intakes adjusted to yield usual intake distribution. This is achieved by removing the variability introduced by the day-to-day variation in B12 intakes within an individual. In this way, the proportion of individuals in a population group at risk of B12 insufficiency can be determined. Details of the adjustment process are given in Chapter 3. In population groups consuming crystalline B12 in fortified foods and in supplements, consumption is often intermittent necessitating several days of intake or use of a food frequency questionnaire. Details of the B12 content of many dietary supplements are available in the U.S. federal Dietary Supplement Ingredient Database. No correction is made for differences in the bioavailability of crystalline B12 from supplements or fortified foods compared with B12 from food because the absorption differences between these two B12 sources are assumed to be small. Alternatively, instead of determining quantitative B12 intakes, estimates of the usual consumption of animal products per day, week, or month can be used to assess risk of inadequate intakes of B12 for individuals in households and for population groups; see Chapter 3 for more details. Several studies have reported significant positive associations between animal source food intake and vitamin B12 status (McLean et al., 2007). A combination of biochemical biomarkers is recommended to assess actual B12 status, together with knowledge of factors such as age, gender, pregnancy, folate status, and bacterial overgrowth, all known to impact some of the B12 biochemical biomarkers. The four priority biochemical biomarkers recommended by the Biomarkers of Nutrition for Development (BOND) project to detect subclinical vitamin B12 deficiency are total serum vitamin B12, serum holotranscobalamin (holo TC), plasma homocysteine, and serum methylmalonic acid; see Allen et al., 2018 for more details. Biochemical assays for each of these biomarkers together with the recommended tests to determine the cause of the vitamin B12 deficiency are outlined below. The BOND project caution that currently no gold standard exists to define vitamin B12‑deficiency using these biochemical biomarkers. If subclinical vitamin B12 deficiency is suspected, then total serum vitamin B12 or serum holoTC is frequently measured first, followed by methylmalonic acid (MMA) or plasma homocysteine (tHcy) concentrations.22b.2.1 Serum vitamin B12
Of the vitamin B12 in the serum, 20‑30% is attached to the transport protein transcobalamin (previously known as transcobalamin II), and the remaining 70‑80% is bound and carried by haptocorrin, the latter historically designated as transcobalamin I and transcobalamin III. Hence, total plasma or serum B12 concentration includes B12 (cobalamin) bound to transcobalamin (holoTC) and B12 bound to haptocorrin. Assessment of total B12 in plasma or serum provides information on intake of B12, long-term B12 status of the individual, and liver stores. Of these two circulating transport proteins, only transcobalamin bound to B12 to form holotranscobalamin (holoTC) in serum is metabolically active, delivering vitamin B12 to receptors on cell membranes. In contrast, because there are no haptocorrin receptors located on most cells, B12 cannot be delivered to extrahepatic tissues by haptocorrin. Moreover, haptocorrin binds not only B12 but also B12 analogs. However, because gastric intrinsic factor does not bind the B12 analogs (only B12), the B12 analogs are not reabsorbed but ultimately excreted in the stool (Renz, 1999). In early vitamin B12 deficiency, when individuals are in negative balance, the amount of vitamin B12 attached to holoTC falls, but there is no concomitant decline in the total serum vitamin B12 concentrations. The latter often remain normal for weeks or months despite low serum holoTC levels. Serum vitamin B12 concentrations only decline when the percentage saturation of total transcobalamin with vitamin B12 falls below 5% (Herbert, 1987). Total serum vitamin B12 concentrations are expressed aspmol/L, or aspg/mL in clinical practice with 1.0pg/mL=0.7378pmol/L. Concentrations above the normal level of 221pmol/L are indicative of an adequate B12 status, whereas those between 150 and 221pmol/L, or often below 150pmol/L, are indicative of B12 depletion or deficiency, respectively. Traditionally, total serum vitamin B12 is often the first-line biochemical test used for routine screening for vitamin B12 deficiency; concentrations reflect both the vitamin B12 intake (Dullemeijer et al., 2013) and body stores. However, to date a single accepted cut‑value for serum B12 indicative of deficiency has not been established. Moreover, the serum B12 test has low sensitivity and specificity and may overestimate B12 deficiency when used alone (Mineva et al., 2021). For example, clinical cases of vitamin B12 deficiency have been reported even in persons with low-to-normal total serum vitamin B12 levels (Lindenbaum et al., 1988). The latter may be explained by the presence of a physiologically inactive proportion of serum B12 being bound to the carrier protein haptocorrin, as explained earlier. Many, but not all of these cases, have shown a positive response to treatment with vitamin B12, based on improvements in abnormal metabolite levels and clinical indices (Stabler et al., 1990). Most available prevalence data on B12 deficiency based on total serum B12 concentrations are focussed on older adults. In reports based on 48 cohorts, 10‑19% of adults more than 60 years of age had total serum B12 levels below 148 or 150pmol/l and said to be indicative of B12 deficiency. A high prevalence of low serum / plasma B12 has also been reported among pregnant women (27.5% in 11 cohorts) and children (12.5% in 14 cohorts) (Smith et al., 2018).Factors affecting total serum vitamin B12
Age influences levels of total serum vitamin B12. Infants and children have higher serum B12 concentrations compared with adults, irrespective of ethnicity, perhaps in part attributed to their higher intake of milk in early childhood and a more efficient hepatic storage of B12 (Abildgaard et al., 2022; Sobczynska-Malefora et al., 2023). During adulthood, serum B12 concentrations tend to decline with age, especially in the elderly (Wahlin et al., 2002), probably arising from the gradual decrease in both gastric acidity and intrinsic factor production that occurs with aging (Asselt et al., 1998; Carmel, 1997). An age-related decrease in the intake of vitamin B12 may be an additional factor (Johnson et al., 2003). However, such a decline in serum B12 among elderly adults is not consistent (Vogiatzoglou et al., 2009; Mineva et al., 2019), possibly due to B12 supplement use in this age group. In the United States NHANES 2011 to 2014 survey, for example, serum vitamin B12 concentrations were reportedly 13% higher in persons aged >70 y compared to those in the 20‑39y age group, a trend attributed mostly to B12 supplement usage (Mineva et al., 2019). Gender may affect serum vitamin B12 concentrations, although results have been inconsistent. In smaller, less well controlled studies levels for men and women have been similar, irrespective of age (de Carvalho et al., 1996; Wahlin et al., 2002). In studies that have controlled for factors known to affect total serum vitamin B12, concentrations were higher in women than in men (Mineva et al., 2019; Metz et al., 1971; Fernandes-Costa et al., 1985), whereas in others no differences between the sexes were found, irrespective of ethnicity (Sobczynska-Malefora et al., 2023). Pregnancy can lead to a 25‑30% fall in total serum vitamin B12 concentrations between 20‑ and 30‑weeks of gestation. The decline is due to decreased synthesis of haptocorrin and hemodilution. Holotranscobalamin (holoTC) concentrations, however, remain unchanged during pregnancy so the decline is unlikely to reflect B12 depletion. Indeed, by 14 weeks postpartum, total serum B12 concentrations are no longer low (Allen et al., 2018). Lactation is accompanied by a 40% increase in serum B12 at 6 weeks post-partum, remaining at this elevated level while the mother is lactating. A corresponding moderate increase in the functional metabolic biomarkers — plasma methymalonic acid and homocysteine — suggests that some depletion of maternal intracellular vitamin B12 has occurred even though serum B12 concentrations are high (Varsi et al., 2018). Ethnicity affects serum/plasma B12 concentrations. People of African origin have higher serum B12 concentrations than other ethnic groups. For example, in the US NHANES 2011-2014 survey, non-hispanic black adults had higher B12 concentrations than non-hispanic white adults, even after controlling for age group and B12 supplement use (Mineva et al., 2019). This ethnic difference is attributed in part to a higher concentration of B12 binding proteins, most notably the transport protein haptocorrin. However, because B12 bound to haptocorrin is not metabolically active, use of only total serum B12 may result in Black patients being incorrectly identified as having a high B12 status. Clearly, failure to apply ethnic-related serum B12 reference intervals will lead to underdiagnosis of B12 deficiency and any associated comorbidities (Sobczynska-Malefora et al., 2023). Folate status, both deficiency and excess, might affect total serum vitamin B12 levels. Folate deficiency may result in moderately low total serum vitamin B12 concentrations because both folate as a cosubstrate (methyl donor) and vitamin B12 (as a coenzyme) are required in the remethylation metabolic pathway of homocysteine (see Figure 22b.2) (Klee, 2000). The mechanism for the interaction between excess folate and B12 is uncertain and warrants further investigation. One proposed hypothesis, as yet untested, is that high-dose folic acid supplements cause depletion of the transport protein holotranscobalamin in serum, thus exacerbating B12 deficiency. Older adults with both low total serum B12 concentrations and increased levels of serum folate appear to have a higher risk of anemia and dementia, based on a review of the clinical and epidemiological evidence. The high serum folate levels are presumed to arise from exposure to high intakes of folic acid, the synthetic form of folate used in fortified foods and supplements (Miller et al., 2024). Bacterial overgrowth of the small intestine may lead to low serum B12 concentrations and vitamin B12 deficiency because the bacteria utilize vitamin B12 for their own metabolism so B12 cannot be absorbed by the body. Fish tapeworm also leads to B12 malabsorption because the tapeworms consume B12 for their own needs, so serum B12 concentrations fall. Chronic infection with Helicobacter pylori, often accompanied by intestinal bacterial overgrowth, may result in low serum B12 concentrations. The latter arise from malabsorption from food-bound B12 induced by the hypochlorhydria associated with atrophic gastritis caused by the H pylori jnfection of the stomach (Sobczynska-Malefora et al., 2021). Lack of gastric intrinsic factor caused by pernicious anemia or gastrectomy leads to low total serum vitamin B12 concentrations. Intrinsic factor (IF), a glycoprotein synthesized by the gastric parietal cells, is required for the absorption of vitamin B12 in the small intestine. In some cases of pernicious anemia, parietal cell auto-antibodies lead to the destruction of IF‑producing parietal cells in the stomach resulting in B12 malabsorption, and thus deficiency. The incidence of pernicious anemia increases with age, and is especially common among persons of European or African descent (Stabler & Allen, 2004). Parietal cell autoantibodies are also found in other autoimmune conditions such as Graves disease, hypothyroidism, and Addison’s disease (Sobczynska-Malefora et al., 2021). Other conditions that produce elevated haptocorrin levels increase total serum B12. Examples of such conditions include myeloproliferative disorders (e.g., chronic myelogenous leukemia, polycythemiavera), severe liver diseases, and alcoholism. In these conditions, although total serum vitamin B12 concentrations may appear elevated or apparently normal, tissue vitamin B12 concentrations will be low (Arendt & Nexo, 2012). Some disease states are associated with low total serum vitamin B12 concentrations because of an inability to absorb or digest protein-bound vitamin B12. Disease states associated with B12 malabsorption due to villous atrophy or mucosal impairment include ileal Crohn’s or Celiac disease, respectively. In disease states such as atrophic gastritis and pancreatic insufficiency, low serum B12 concentrations arise because digestion of protein-bound B12 is compromised. Other intestinal diseases such as tropical sprue, intestinal lymphoma, amyloidosis, and short bowel syndrome have also been associated with low serum B12 levels and B12 depletion. Surgery involving partial or complete removal of any part of the gastrointestinal tract, including some bariatric surgery procedures, will reduce food-bound B12 absorption and lower serum B12 concentrations. Inborn errors of intracellular B12 metabolism can result in low total serum B12 levels through their effects on absorption, transport, or metabolism of B12. The genetic defects range from rare gene deletion and mutations to single nucleotide polymorphisms with more mild and subtle effects. In congenital pernicious anemia, low serum total B12 concentrations arise from B12 malabsorption caused by mutations in a gene (GIF,CBLIF) and defects in the synthesis of intrinsic factor. The rare autosomal recessive disorder Immerslund-Grasbeck (IGS) disease is caused by mutations in either the gene Amnion Associated Transmembrane Protein (AMN) or Cubilin (CUBN) gene responsible for the synthesis of cubam receptors (Sobczynska-Malefora et al., 2021). Genetically predetermined deficiencies of the two B12 transport proteins, congenital transcobalamin deficiency (gene locus 22q11.2-qter) or haptocorrin deficiency (gene locus 11q11-q12), can be associated with total serum B12 values that may be normal, or falsely low, respectively (Amos et al., 1994). Individuals with defects of methylmalonyl CoA mutase or polymorphisms occurring in the genes that code for enzymes in the cobalamin adenosylation pathway, may also have low serum vitamin B12 levels (Kano et al., 1985). Eight genetic defects in B12 metabolism have been identified all of which result in a failure to utilize B12 by the target cells; for more details see Sobczynska-Malefora et al. (2021). Restricted dietary intake of B12 is associated with veganism and to a lesser extent vegetarianism, dietary patterns that avoid all or certain animal-source foods, respectively. Vegans have low total serum B12 concentrations unless crystalline B12 from fortified foods or supplements are consumed. To date, how long it takes for vitamin B12 deficiency to develop when individuals adhere exclusively to a fully plant-based diet is unknown. There have been several reports of low plasma vitamin B12 levels among Asian Indians in Great Britain, the United States, and the Indian Subcontinent, many of whom are likely to practice vegetarianism so intake of animal-source foods is low (Antony, 2003; Antony, 2001; Carmel et al., 2002). Several investigators have reported an inverse relationship between intake of animal-source foods and total serum vitamin B12 levels. In a study in Germany of omnivores and vegetarians, none of whom were taking vitamin supplements, those consuming omnivorous diets had higher serum B12 concentrations than the lacto‑ or lacto‑ovo vegetarians both of whom avoided meat, poultry, and fish, who in turn had higher levels than the vegans (Herrmann et al., 2003). Similar trends in serum B12 concentrations have been reported among British male omnivores, vegetarians, and vegans in the EPIC Oxford cohort study (Gilsing et al., 2010). Dietary intakes of omnivores are not generally strongly related to serum vitamin B12 levels; low correlations in daily B12 intakes have been linked to the large size of liver vitamin B12 stores (2‑3mg) (Doets et al., 2013). A meta-analysis of randomized controlled trials (RCTs) and observational studies investigated the dose-response relationship between vitamin B12 intake and serum or plasma vitamin B12 concentrations. Doubling vitamin B12 intake was estimated to increase the vitamin B12 concentration in serum or plasma by 11% (95% CI: 9.4%, 12.5%) (Dullemeijer et al., 2013). Several cross-sectional studies among healthy adults with normal absorption of B12 show that serum B12 concentrations reach a maximum plateau and stabilize at B12 intakes of 4‑7µg/d (Bor et al., 2010). Mode of infant feeding affects total serum B12 levels; infant formula has a higher B12 content than human milk (Greibe et al., 2016). Vitamin B12 supplement use increases serum B12 concentrations. In the US NHANES 2011‑2014 survey, serum B12 concentrations for adults were about 40% higher in crystalline B12 supplement users than nonusers overall (i.e., for age groups 20‑39, 40‑59, 60‑69, and >70 years), with the greatest increase among older persons. In non‑ supplement users, however, serum B12 concentrations were similar across all four age groups (Mineva et al., 2019). Medications such as histamine 2 receptor antagonists and proton pump inhibitors (PPIs) (lansoprazole, omeprazole, and esomeprazole) that suppress gastric acid may lower total serum B12 levels by impairing the release of B12 from food (Damodharan et al., 2021; Mumtaz et al., 2022). Metformin, used as treatment for type 2 diabetes, induces B12 malabsorption and has also been associated with low total serum B12 concentrations (Al‑Fawaeir & Al‑Odat, 2022). However, the low serum B12 levels may not reflect true B12 deficiency in view of the finding in animal studies that metformin increases liver accumulation of vitamin B12 (Greibe et al., 2013; Obeid et al., 2024). Storage of blood samples prior to separation of the serum affects B12 concentrations if separation is delayed. Such delays can lead to an initial rise in total serum B12 concentrations, followed by a significant drop after 1 to 3 days (Allen et al., 2018). Hence to ensure accurate measurements, it is important to separate the blood samples and freeze the serum as soon as possible after collection. Analytical method may affect the reported serum vitamin B12 concentrations. Some of the nonfunctional analogs of vitamin B12, as well as the cobalamins, were measured in earlier radio-assays. This led to overestimates of actual serum B12 concentrations. This problem has now been overcome by using purified intrinsic factor which does not react with vitamin B12 analogs as the binder in the immune-enzymatic assays (Klee, 2000). However, in patients with pernicious anaemia these assays should not be used because the intrinsic factor antibodies in the patient's serum interferes with the binding of B12 to the intrinsic factor used in the assay, generating spurious results. For the analytical platform used it is essential to adopt reference ranges that are compatible with the chosen platform (Harrington, 2017). A standard reference material for serum B12 has been developed by the US National Institute for Standards and Technology (NIST) (SRM 3951). This SRM consists of three sera with differing vitamin B12 concentrations: 100pg/mL; 200pg/mL; 450pg/mL.Interpretive criteria
Interpretation of serum B12 concentrations deemed as adequate or inadequate is challenging. The normal levels for serum vitamin B12 concentrations in healthy persons are affected by many factors unrelated to vitamin B12 status, some of which are not considered when interpreting the results. These factors may include age, ethnicity, pregnancy, use of certain medications, individual genetic variation, the assay method and any measurement errors associated with its use. All these factors may affect the sensitivity and specificity of serum B12 as a biomarker of B12 status and result in false-positive or false-negative classification of vitamin B12 deficiency. Traditionally, adequate concentrations of biomarkers such as serum B12 are defined by a reference interval which covers 95% of the results obtained in a healthy, non-diseased population. In practice, however, it is difficult to compile a “true” healthy reference and few exist for serum B12. Two methods are used to define whether a biomarker is deemed “inadequate”, both of which have been employed for serum B12 concentrations. In some studies, a serum B12 concentration is considered inadequate when it falls below a statistically defined lower reference limit, often based on the 2.5th percentile value from an “apparently healthy” reference distribution compiled within a country. Hence, values for serum B12 that represent the 2.5th percentile will vary, depending on the local reference distribution applied. Alternatively, the diagnostic criteria used to identify inadequate B12 status have been based on a known relationship between the serum B12 biomarker and low body stores, functional impairment, or clinical signs of deficiency. In such cases, the term “cutoff” should be used. In practice, the term “cutoff” is often used when the value for inadequate B12 has been based on a statistically defined reference limit. Again, cutoffs for serum B12 designated in this way can also vary, depending on both the local conditions and adverse outcome applied. Frequently, the cutoffs for serum B12 indicative of inadequacy have been set based on the relationship between serum B12 and serum methylmalonic acid. The latter is a functional biomarker considered to be relatively specific and sensitive biomarker of B12 status. For more discussion on the Evaluation of nutritional assessment indices, see Chapter 1: Introduction Section 1.6. Clearly, the reference limit or cutoff applied to designate inadequate vitamin B12 status may vary, leading to differences in the literature on reports for the prevalence estimates for vitamin B12 deficiency. Reports on the various methods used to interpret serum B12 concentrations as adequate or inadequate are outlined below. The United Kingdom compiled the mean, median, and critical lower and upper percentiles for serum B12 concentrations by sex and age for children, young people aged 4 to 18y, and people aged >65y based on data from the U.K. National Diet and Nutrition Surveys (Gregory et al., 1995; Gregory et al., 2000; Finch et al., 1998). More recently, Kerr et al. (2009) have proposed age-specific 5th and 95th percentile reference ranges for serum B12 based on data from a representative sample of British children aged 4 to 18y from the National Diet and Nutritional Survey. Serum B12 concentrations decreased significantly with age, with values for those consuming fortified breakfast cereals being significantly higher than non-consumers, a trend that was independent of age and gender. Up to aged 14 years, the 5th and 95th percentiles of serum B12 concentrations were 242‑749pmol/L for children aged 4‑10 years (n = 317) and 172‑641pmol/L for children aged 10‑14 years (n = 263). For children from aged 15‑18 years, sex specific 5th and 95th percentiles were compiled. These values were 139‑452pmol/L for boys (n = 113) and 108‑502pmol/L for girls (n = 132) (Kerr et al., 2009). Serum vitamin B12 assays in this survey were conducted on a semiautomated analyzer using microparticle enzyme immunoassay (MEIA) technology (Kerr et al., 2009). In the United States, 95% reference intervals for serum B12 concentrations for four age groups have been reported based on data from apparently healthy adults aged 20 > 70 years from NHANES 2011 to 2014 (Mineva et al., 2019). These are shown in Table 22b.1.Age group | Serum vitamin B12, Median (95% CI,pmol/L) | Serum vitamin B12, 5th‑97.5th percentile |
---|---|---|
All | 378 (372, 384) | 158‑1140 |
20‑39 | 373 (364, 381) | 167‑840 |
40‑59 | 365 (357, 378) | 160‑1150 |
60‑69 | 398 (375, 420) | 152‑1530 |
≥70 | 416 (401, 428) | 137‑1720 |
Age group (y) | 95% RI, B12 (pmol/L) | % of B12 patient samples below/above 95% RI |
---|---|---|
0 ‑ <1 | 180‑1400 | 3.3%/0.0% |
1 ‑ <12 | 260‑1200 | 5.5%/2.1% |
12 ‑ <18 | 200‑800 | 2.8%/3.4% |
18 ‑ <65 | 200‑600 | 3.0%/12.1% |
≥65 | 200‑600 | 2.2%/19.2% |
Age groups (y) | 0‑1 | 2‑5 | 6‑9 | 10‑13 |
---|---|---|---|---|
Data size (N) | 105 | 474 | 492 | 661 |
95 % CI Lower Limit of RI | 142–178 | 246–308 | 221–270 | 168–207 |
RIs (pmol/L) | 159–1025 | 276–1102 | 245–798 | 187–643 |
95 % CI Upper Limit of RI | 971–1081 | 1042–1165 | 750–848 | 593–697 |
Age groups (y) | 14–17 | 18–29 | 30–39 | 40-49 |
Data size Black (N) | 267 | 1038 | 1534 | 1983 |
95 % CI Lower Limit of RI Black | 152–191 | 146–175 | 150–186 | 161–198 |
RIs Black (pmol/L) | 171–639 | 160–632 | 167–730 | 178–807 |
% Upper Limit of RI Black | 585–697 | 579–691 | 665–801 | 739–882 |
Data size Asian/White (N) | 297 | 2611 | 3614 | 3390 |
95 % CI Lower Limit of RI Asian/White | 135–165 | 119–148 | 126–161 | 127–160 |
RIs Asian/White (pmol/L) | 149–456 | 133–458 | 143–506 | 142–501 |
95 % CI Upper Limit of RI Asian/White | 420–494 | 416–505 | 460–555 | 458–548 |
Serum B12 (pmol/L) | Serum
holotrans- cobalamin (pmol/L) | Serum methyl- malonic acid (nmol/L) | ||||
---|---|---|---|---|---|---|
Deficient | Adequate/ Normal | Deficient | Adequate/ Normal | Deficient | Adequate/ Normal | |
Newborn cord blood | 120-690 | 33-240 | 170-500 | |||
6 mo | 121-520 | 12-90 | 140-220 | |||
12 mo | 165-580 | 19-100 | 120-830 | |||
24 mo | 183-260 | 29-110 | 120-300 | |||
Adults | Severe <75 Deficient 75 - <150 Depleted 150-221 | >221 | <35-40 | 40-150 or 40-200 | >376 or >271 |
Measurement of serum vitamin B12
Initially serum B12 was measured by microbiological methods, using bacteria with relatively specific requirements for vitamin B12 for growth. Examples include both Lactobacillus delbrueckii subsp. lactis (ATCC 4797), also known as L. leichmannii, or Euglena gracilis (Herbert et al., 1984). The microbiological assay has several limitations. For example, bacteriostatic substances in the blood, including antibiotics or cancer chemotherapeutic agents, inhibit the growth of the microorganism and interfere with the assay, producing misleadingly low serum vitamin B12 concentrations. The main disadvantage of the microbiological method, however, is its low specificity as it measures a variety of cobalamin analogs that are not necessarily biologically active (Sobczynska-Malefora et al., 2021). Competitive-binding assays that use radioisotope dilution methods for detection are simpler and less time consuming than the microbiological assays. Many of the available competitive-binding assays methods have been automated (Chan, 1996). and unlike the microbiological assays, they are not affected by antibiotics or cancer chemotherapeutic agents. The coefficient of variation among the six laboratories for serum vitamin B12 analyzed by automated competitive-binding assays has been reported to vary from 4.4% to 10.0% (Klee, 2000). However, the method requires the addition of radioactive cyanocobalamin to compete with vitamin B12 in the serum for the binding sites on an added cobalamin-binding protein. Purified hog intrinsic factor is now often used as the binding protein. Alkaline conditions are used to disrupt the vitamin B12 from the binding proteins, after which the vitamin B12 is generally converted to cyanocobalamin by potassium cyanide, prior to measurement (Klee, 2000). However, the assay is falling into disuse with possible discontinuation of the kits (Allen et al., 2018). More recently, clinical laboratories are using high throughput automated competitive binding chemiluminescence assays (CBLA), using purified intrinsic factor as a reagent to measure total vitamin B12 after its release from endogenous binding proteins. For this method both samples and comparators should be protected from light during collection and separation. Caution should be observed when this method is used to interpret results in patients with pernicious anemia. In these patients some of the competitive binding chemiluminescence assays can be influenced by the presence of interfering anti‑IF antibodies, thereby providing spuriously elevated serum cobalamin concentrations, as noted earlier. Serum B12 can also be determined using B12‑antibodies and B12 enzyme-linked immunosorbent assay (ELISA) kits. Various kits are available. Although these could potentially provide an alternative to IF‑based assays, they have not been completely verified (Sobczynska-Malefora et al., 2021). Both serum and plasma samples in which EDTA has been used as the anticoagulant can be used for all the B12 analytical methods. However, use of lithium heparin should be avoided, as this anticoagulant may produce gelatinous serum and elevated B12 concentrations. Blood samples for analysis of serum B12 should be protected from light during collection, and whole blood separated as soon as possible as delays in centrifugation and separation can lead to an initial rise in serum B12 concentrations (Allen et al, 2018).22b.2.2 Serum holotranscobalamin
Serum holotranscobalamin (holoTC) is the physiologically active metabolite of vitamin B12 that delivers the vitamin to all DNA synthesizing cells, as discussed earlier. Only 20‑30% of the total vitamin B12 in serum is bound to the transcobalamin protein. Holo‑TC accounts for 5‑20% of total transcobalamin which is made in the ileal enterocytes from intracellularly synthesized transcobalamin and absorbed vitamin B12. Serum holoTC, like serum B12, reflects a broad range of intakes and status (Yetley et al., 2011), but is more sensitive and specific than serum B12. For example, because holoTC has a short half-life, concentrations quickly fall below normal after vitamin B12 absorption ceases. Hence, low holoTC concentrations in serum are often considered the earliest indicator of negative vitamin B12 balance. With increasing intakes of vitamin B12, concentrations of holoTC rise continuously at least until vitamin B12 saturates the transport proteins. The holoTC assay is unaffected by interference from high-titre intrinsic factor antibody levels. Moreover, serum holoTC is not subject to the 25‑30% fall in pregnancy that is bserved with serum B12. At postpartum, serum B12 rises substantially. Instead, serum holoTC increases during pregnancy, a trend that continues to 6 weeks post-partum. Thus, in pregnancy serum holoTC offers a diagnostic advantage over total serum B12 (Varsi et al., 2018). Serum holoTC is also a useful test for identifying patients who may suffer from suspected deficiencies in the two vitamin B12 binding proteins, transcobalamin and haptocorrin. Serum holoTC is a component of the CobaSorb test now used as a surrogate for the dual isotope Schilling test (Nexo & Hoffman-Lucke, 2011) (see Section 22b.2.7). In the past the Schilling test was the gold standard method to evaluate the functional capacity of the ileal IF‑B12 receptor and required the use of cobalt-labeled B12. However, the Schilling test is no longer available worldwide due to safety factors related to use of radioactive B12 and is now considered obsolete.Factors affecting serum holotranscobalamin
Age, sex, and race and their effects on serum holoTC concentrations appear limited. Only a few reports are available with inconsistent results (Nexo & Hoffman-Lucke, 2011). Hence, currently data are insufficient to take into account the slight differences in serum holoTC concentrations by age, sex, and possibly race for adults. Pregnancy does not affect serum holoTC, levels increasing from 18 weeks gestation to 6 weeks postpartum. This trend contrasts with the decline in serum B12 observed during pregnancy (Varsi et al., 2018). The latter results from a decrease in the synthesis of haptocorrin and possibly hemodilution. Consequently, assay of serum holoTC rather than serum B12 is preferable in studies of pregnant women. Inherited defects such as transcobalamin deficiency is associated with unmeasurable levels of holoTC in serum. This genetic defect is associated with severe B12 deficiency because lack of the trancobalamin protein prevents B12 binding and formation of holoTC. Early treatment, however, can lead to a good clinical outcome. Presence of the rare minor allele re35838‑82 (p.R215W) in the transcobalamin gene is also associated with unmeasurable levels of holoTC in serum. In this defect, there is proportionately more B12 bound to serum haptocorrin, the physiologically inactive B12 transport protein. This genetic defect is more common in South Asians and those of African origin, and unlike transcobalmin deficiency, is associated with other B12 biomarkers in the normal range and no clinical symptoms. Transcobalamin receptor (TCblR/CD320) polymorphisms may also impact on serum holoTC concentrations. In such cases, proportionately more B12 is bound to holoTC leading to elevated serum holoTC levels. Therefore, in such rare conditions (present in 5% of older adults), holoTC might not be a marker of "true" intracellular B12 (Sobczynska-Malefora et al., 2021). Disease conditions affecting serum holoTC levels include renal patients with impaired kidney function. With decreasing kidney function, levels of serum holoTC rise to ensure sufficient holoTC is delivered into cells. Therefore, in patients with renal insufficiency (assessed by measurement of serum creatinine), serum holoTC concentrations may appear normal, and are no longer a useful biomarker to predict B12 status (Herrmann et al., 2005). Other conditions that might elevate serum holoTC concentrations include liver diseases and possibly the development of autoantibodies against transcobalamin. High folic acid intake has been associated with reduced serum holoTC concentrations. This adverse effect on B12 status has only been implicated with synthetic folic acid and not with natural folate from food sources (i.e., methyltetrahydrofolate) (Selhub et al., 2022). However, in countries with mandatory folic acid fortification without B12 fortification, a high-folate-low vitamin B12 interaction may be cause for concern (Sobczynska-Malefora et al., 2021). Dietary B12 intakes affect serum holoTC concentrations with, as expected, lower levels in vegans than omnivores due to their lower B12 intake. Serum holoTC is a better biomarker of recent B12 intake than serum B12 (or methymalonic acid or tHcy) because serum holoTC concentrations increase much faster after ingestion of B12 from a meal (i.e., 6 hours) or crystalline B12 from supplements.Interpretive criteria
Available serum holoTC assay methods, unlike serum B12, give similar values according to a European comparison study (Morkbak et al., 2005). However, to date there is insufficient data to account for the slight differences in serum holoTC concentrations by age, sex, and possibly race for adults. Furthermore, uncertainty exists about the appropriate serum holoTC cutoff to diagnose B12 deficiency. For adults, the cutoff for serum holoTC, based on the relation of holoTC to serum methylmalonic acid, is <35‑40pmol/L. according to the BOND group. They provide no cutoff for deficiency for children. However, for early childhood, the BOND group set tentative normal reference ranges for four age groups from birth to 24 months of age; see Table 22b.4 (Allen et al., 2018). Currently the BOND group have set a tentative range indicative of adequate/normal B12 status of 40‑150 or 40‑200pmol/L for healthy adults, irrespective of age and the assay used. A summary of reference intervals for serum holoTC using the most common assays are shown in Table 22b.5.Method | Sample (µL) | Reference range (pmol/L) | n | Reference |
---|---|---|---|---|
1 Radio- immunoassay | 400 | 24–160 37‑170 | 105 303 |
Ulleland et al. (2002)
Loikas et al. (2003) |
2 Microbiology | <150 | 42–160 | 500 | Refsum et al. (2006) |
3 ELISA | 100 | 40‑150 | 137 | Nexo et al. (2002) |
4 Direct | 200 | 19–130 36‑220 | 292 276 |
Brady et al.(2008)
Aarsetøy et al. (2008) |
Measurement of serum holo transcobalamin
Several methods are available for measuring serum holoTC. They include a radioimmunoassay (RIA) method based on monoclonal antibodies against transcobalamin (Vu et al., 1993) and an enzyme-linked immunosorbent assay procedure that is easier to use and measures holoTC directly without sample pretreatment (Brady et al., 2008). The holoTC‑RIA method has now been replaced by an assay that uses holoTC-specific monoclonal antibodies and yields results comparable to those of the holoTC‑RIA. An automated assay has been developed for this method using the Abbott AxSYM immunoassay analzyer (Brady et al., 2008). Tests have confirmed that results based on the holoTC‑RIA and the holoTC-enzyme-linked immunosorbent assay yield similar values (Morkbak et al., 2005). Blood sampling and storage conditions have limited effect on serum or plasma holoTC concentrations. Therefore, no special precautions are necessary for drawing blood samples and concentrations are stable in serum stored at −20°C to −70°C for at least 16 months. Both serum and plasma can be used for the assay. Fasting and non-fasting blood samples can be used because holoTC concentrations are unaffected by the intake of a normal diet (Nexo & Hoffman-Lucke, 2011).22b.2.3 Deoxyuridine suppression test
This sensitive in vitro test has been used to diagnose early vitamin B12 (or folate) deficiency even in the absence of morphological changes in the blood. It was developed by Killman (1964) and Metz et al. (1968). Abnormal deoxyuridine suppression is the biochemical expression of disordered DNA metabolism. Although either lymphocytes or whole blood can be used for this test to detect past vitamin B12 or folate status, generally bone marrow cells are preferred because they measure the acute status (Colman 1981). The test is rarely used today because it requires bone marrow cultures, uses a radioactive label, is difficult to control (Carmel et al., 1996; Chanarin & Metz, 1997), and is not specific to vitamin B12 deficiency.22b.2.4 Serum methylmalonic acid
Methylmalonic acid (MMA) accumulates in the serum or plasma when the supply of vitamin B12 is reduced; it does not rise in folate deficiency (Savage et al., 1994). Hence, the measurement of methylmalonic acid in serum or plasma, is a sensitive and specific marker of tissue vitamin B12 deficiency, reflecting stores rather than intake. Concentrations are elevated in vitamin B12 deficiency, even in the absence of clinical signs or symptoms or of morphological changes in the blood. For example, in a cross-sectional study of 2919 Dutch elderly people with elevated serum homocysteine (tHcy) levels (i.e., >12 mol/L), the association of total serum B12 levels with serum MMA (and tHcy) was explored with restricted cubic splines (Figure 22b.5).
Macrobiotics (n = 41) | Controls (n = 50) | Significance | |
---|---|---|---|
MMA (µmol/L) | 1.44 (0.17‑12.15) | 0.18 (0.06‑0.51) | p < 0.0001 |
Hcy (µmol/L) | 13.5 (6.8‑26.8) | 7.59 (5.3‑11.0) | p < 0.0014 |
Cobalamin (pmol/L) | 141 (59‑340) | 399 (194‑821) | p < 0.0001 |
Cysteine (µmol/L) | 164 (119‑226) | 184 (145‑233) | p = 0.0001 |
Methionine (µmol/L) | 6.0 (1.5‑23.8) | 6.9 (1.1‑41.9) | p = 0.44 |
Serum level | Prevalence in cobalamin deficient patients (%) | Prevalence in folate deficient patients (%) |
---|---|---|
Elevated MMA | 98 | 12 |
Elevated Hcy | 96 | 91 |
Elevated MMA, Hcy normal | 4 | 2 |
Elevated Hcy, MMA normal | 1 | 80 |
Hcy and MMA both normal | 0.2 | 7 |
Factors affecting serum methymalonic acid
Age tends to increase serum MMA concentrations, particularly in persons >70y. This trend was reported in the US NHANES 2013‑2014 survey of adults >20 years, with levels about 60% higher in those aged >70y compared to the youngest age group (i.e., 20‑39 y) (Mineva et al., 2019). It is of interest that this age-related trend in serum MMA levels was apparent even among persons in the NHANES 2013‑2014 survey with serum total B12 concentrations indicative of replete B12 status (i.e., >300pmol/L) and with normal renal function, as shown in Table 22b.8.Age group (y) | Sample size | Median (95% CI) | 2.5th‑97.5th percentile |
---|---|---|---|
All | 5481 | 130 (127, 133) | 67.2‑281 |
20‑39 | 2178 | 120 (118, 124) | 63.5‑254 |
40‑59 | 1988 | 133 (128, 138) | 70.5‑293 |
60‑69 | 824 | 143 (134, 149) | 72.4‑281 |
≥70 | 491 | 161 (151, 167) | 84.3‑317 |


Interpretive criteria
Currently there is no consensus for the cutoff value for serum MMA acid concentrations indicative of vitamin B12 deficiency. Two tentative “cutoffs” for deficiency in adults are presented in Table 22b.4 from the BOND group (Allen et al., 2018). These so called cutoffs are derived from serum MMA concentrations compiled from US NHANES 2003‑2006 data in which the central 95% reference intervals (2.5th to 97.5th percentile) for serum MMA concentrations were presented (Pfeiffer et al., 2013). Bailey et al. (2013) argues that use of only one serum MAA cutoff point can result in those with a B12 status considered intermediate being misclassified into the sufficient and deficient groups. To resolve this uncertainty, these investigators examined the complex relation between serum B12 and MMA (adjusted age, glomerular filtration rate, and hours of fasting) based on data from 12,683 adult participants of the NHANES 1999‑2004 study. Based on statistical modeling, those participants with a serum B12 <126pmol/L had the highest serum MMA (ie., median 281nmol) whereas for those with a serum B12 >287pmol/L (who likely had adequate B12 status), serum MMA was low (i.e., 120nmol/L). They also identified an intermediate group (i.e., between those with severe B12 deficiency and those with optimal vitamin B12 status) who were defined by a serum MMA of 148nmol/L, although their vitamin B12 status was difficult to interpret. A range of adequate/normal serum MAA concentrations for infants and children up to 2y are also provided by the BOND group (See Table 22b.4). These values are from the same sample of well-nourished breastfed Norwegian infants used to set the age-specific adequate/normal values for serum B12. Data from the US NHANES 2011‑2014 survey have been used to establish 95% reference intervals for serum MMA concentrations by age for US adults >20y. Reference intervals for serum MMA based on the overall sample are available and also on a subpopulation with serum B12 values said to be indicative of B12 repletion (i.e., ≥300pmol/L) and normal renal function based on serum creatinine (See Table 22b.8). Pregnant and lacating women were excluded in both datasets. Not surprisingly, these age-specific central 95% reference intervals established for healthy adults (i.e., with serum B12 >300pmol/L and normal renal function) are lower than the upper‑end MMA cutoffs in earlier reports whose participants were from "apparently healthy populations" but with an unknown clinical history. In these earlier reports, some older participants probably had impaired renal function that was not considered. Clearly, in studies designed to establish adequate/normal serum MAA adult values, it is advisable to evaluate renal function using serum creatinine, especially in older adults. Note that if both serum methylmalonic acid and homocysteine are analysed, a distinction can be made between vitamin B12 and folate deficiencies. Elevated levels of both metabolites are expected in vitamin B12 deficiency, whereas in folate deficiency, only increases in serum homocysteine concentrations occur.Measurement of serum methylmalonic acid
The analytical procedures most frequently used for serum methylmalonic acid involve liquid chromatography-tandem mass spectroscopy (LC‑MS/MS). Advantages of these procedures include the small sample size needed, their high throughput, and good precision. A summary table of the characteristics of the differing LC‑MS/MS procedures available is presented in Jin et al. (2022). In some cases, the LC‑MS/MS procedure has been used to analyze MMA in serum, urine, or dried blood spots. The major drawshy;back of the LC‑MS/MS procedures is their cost, the availability and maintenance of the equipment, and expertise required (Allen et al., 2018). A more cost-effective method requiring small volumes of reagents with high throughput has been developed for serum MAA using stable-isotope-dilution LC‑MS/MS. Application of this method will facilitate early identification of vitamin B12 deficiency among the elderly (Jin et al., 2022). Levels of MMA in serum are stable for several years, when samples are stored at −70°C.22b.2.5 Urinary methylmalonic acid excretion
Vitamin B12 serves as a cofactor for methylmalonyl CoA mutase (EC 5.4.99.2) as noted earlier.This enzyme acts in the conversion of methylmalonyl CoA to succinyl CoA (Figure 22b.7). Therefore, in vitamin B12 deficiency, methylmalonic acid accumulates in the blood and is excreted by the kidneys in increased amounts in the urine, resulting in methylmalonic aciduria (Norman & Morrison, 1993). The biomarker methylmalonic acid in urine is sensitive, detecting early functional vitamin B12 deficiency before the onset of macrocytic anemia, as noted for MMA in serum. Similarly, this test is also highly specific and is not affected by folate deficiency. Only rare congenital enzyme defects discussed earlier (i.e., methylmalonic aciduria) interfere with the test. In elderly patients with renal disease, methylmalonic acid accumulates so levels in urine, like serum, are elevated leading to a decreased specificity for B12 deficiency. In such cases, MMA concentrations in urine should be adjusted for creatinine excretion (Norman & Morrison, 1993) and the ratio of urinary MMA to creatinine (uMMA/C) applied to assess B12 status. Such an approach is recommended in view of the finding that uMMA/C is not dependent on renal function (Supakul et al., 2020). Normal levels for serum creatinine range from 0.6‑1.1mg/dL for women and 0.7‑1.3mg/dL for men (Allen et al., 2018).Interpretive criteria and measurement of methylmalonic acid in urine
The reduced specificity of plasma MMA during renal impairment has led to the development of the uMMA/C ratio in the diagnosis of B12 deficiency. A threshold for uMMA/C ratios of 1.45µmol/mmol has been established for adult patients with plasma B12 concentrations in the low-normal range (i.e., 201‑350ng/L). This threshold has good diagnostic performance for B12 deficiency in patients with subnormal serum or plasma B12 concentrations and renal impairment (Supakul et al., 2020). Assay of urine for MMA avoids the need for blood collection, although excretion of urinary MMA increases after meals. Analysis of 24h urine samples avoids this problem, but collection of 24hr urine samples is hard to achieve. Instead, analyses of two casual urine samples to correct for variability in the MMA concentration in urine can be used. Combined gas/ liquid - chromщatography mass-spectrometry techniques (LC‑MS/MS or GC‑MS/MS) are used to measure MMA in urine, as discussed for serum MMA. They are sensitive and reliable (Norman & Morrison, 1993; Allen et al., 2018) but the procedure is technically difficult; the equipment is expensive, needs high maintenance and expertise. The test is best performed on a 24h urine sample. Recently a method has been developed and validated in which MMA/creatinine can be assayed in fasting urine samples collected on filter paper. After eluting the dry urine samples from the filter paper with a solution containing internal standards followed by filtration, the MMA/creatinine ratios are measured by ultra-performance LC‑MS/MS. The dried urine samples are stable at 86 days at room temperature and can be used for screening B12 deficiency in the elderly (Boutin et al., 2022). Methylmalonic acid in urine is stable at room temperature, provided concentrated hydrochloric acid is added to the urine sample, and for several years, when urine specimens are frozen at −70°C.22b.2.6 Red blood cell methylmalonic acid
Unrecognized deficiency of vitamin B12 during early childhood can lead to long-term health outcomes in children including impaired cognitive function and developmental delay. Maternal B12 deficiency during pregnancy is the most common cause of vitamin B12 deficiency during early infancy, as both B12 fetal stores and vitamin B12 concentrations in breast milk are low. During later infancy and early childhood inadequate intakes of B12 due to low intake of animal-source foods and possibly malabsorption, may play a role (Sobczynska-Malefora et al., 2023). Methylmalonic acid is considered the most specific functional biomarker and a sensitive indicator for B12 deficiency, as noted earlier. In many countries, analysis of dried blood spots are used as a diagnostic tool for newborn screening. In many countries, however, even though dried blood spots are collected routinely from newborns, the blood spots are not routinely screened for B12 status. Therefore, developing a sensitive assay of MMA in dried blood spots through a newborn screening programme has the potential to facilitate the timely diagnosis and treatment of neonatal B12 deficiency. Such a method in dried blood spots could also facilitate testing B12 status in large-scale surveys and in remote populations (Schroder et al., 2014). Details of the assay of MMA in dried bloods spots and their interpretation are given below.Interpretive criteria and measurement of methylmalonic acid in dried blood spots
A new highly sensitive procedure based on stable isotope dilution with liquid chromatography-tandem mass spectrometry (LC‑MS/MS) has been used to establish a reference interval for MMA in dried blood spots for healthy term newborn infants. Details of the method are available in Schroder et al. (2014). The mean dried blood spot MMA concentration quantified from 160 newborns was 16.8pmol/L (95th CI:15.9‑17.6pmol/L per 8‑mmspot), with 95% reference intervals (2.5th to 97.5th percentile) for MMA of 9.89 to 29.3pmol/8‑mm blood spot (0.450 to 1.33µmol/L whole blood). Concentrations of MMA above the upper limit of this reference interval indicate an increased risk of B12 deficiency. The establishment of this neonatal MMA reference interval in dried blood spots will assist in the timely diagnosis and treatment of neonatal B12 deficiency through newborn screening programmes. To date, comparable reference intervals for MMA in dried blood spots of adults have not been established. Concentrations of MMA in dried blood spots increase with storage at room temperature so care must be taken to store dried blood spots below −80°C prior to analysis. Nevertheless, because storage at room temperature for at least one week has no effect on the dried blood spot MMA concentrations, they can be shipped at room temperature (Schroder et al., 2016).22b.2.7 Measurement of vitamin B12 absorption
Once vitamin B12 deficiency has been diagnosed, it is often of interest to establish if malabsorption is the cause. Originally, the Schilling test was used for this purpose, but it is no longer used in view of the safety factors related to the use of radioactive B12 (57Co‑cyanocobalamin) (Sobczynska-Malefora et al., 2021). Instead, alternative tests are now employed that measure either the change in serum holoTC (CobaSorb) concentrations or the absorption of microbially produced cobalamin labeled with 14Carbon or 13Carbon. However, the tests employing both 14Carbon or 13Carbon labeled cobalamin require more validation to diagnose B12 malabsorption in clinical patients (Miller & Green, 2020). CobaSorb test depends on the fact that newly absorbed B12 circulates in the plasma as holoTC. The test is used to clarify whether the B12 deficiency diagnosed in a person is caused by an inability to absorb the vitamin. It is important that the test is performed before participants are treated for B12 deficiency in order to detect an increase in serum holoTC. The test is based on the analysis of serum holoTC prior to and following an oral dose of unlabeled B12. For the test, first a blood sample is taken to measure plasma holoTC, after which a dose of 9µg of crystalline B12 in water is given orally at 6h intervals over a 24h period. The next day, a second blood sample is taken, so that the change in the amount of B12 bound to serum holoTC can be determined. A small or no increase in holoTC is indicative of malabsorption. The test measures relative absorption and does not provide a quantitative estimate of B12 bioavailability Brito et al., 2018). Both the limited use of holoTC assays and lack of interpretative knowledge in many clinical settings have prevented widespread use of the CobaSorb test. 14C-labeled B12 absorption test uses carbon‑14-labeled vitamin B12 (14C-B12) produced by growing a genetically modified strain of Salmonella enterica in a medium containing 14C dimethybenzimidazole. After oral ingestion of 14C‑B12, enrichment of 14C in blood, urine, and stool samples can be measured using accelerator mass spectrometry. This approach has been used to assess absorption and bioavailability of B12 from aqueous solutions as well as some endogenously enriched foods. Exposure to radioactivity is negligible because the enrichment levels of 14C‑B12 can be measured in very small amounts (microliters) of the biological samples (Carkeet et al., 2006). 13C-labeled B12 absorption test is a nonradioactive method for determining vitamin B12 absorption and bioavailability. 13C‑cyanocobalamin is a safe stable isotope-labeled vitamin B12 also biosynthesized from Salmonella enterica (Devi et al., 2020). For this test, a baseline blood sample followed by serial blood samples between 5 and 7h post oral administration of the 13C‑cyanocobalamin are required. After processing, enrichment of plasma concentrations of 13C‑cyanocobalamin is assessed at 5 and 7h post dose by ultra-HPLC-MS. The kinetics of the plasma appearance of 13C‑cyanocobalamin in a 2‑compartment model provide an index of oral absorption and bioavailability of B12. Both the 14C and 13C labeled absorption tests show promise but have not yet been developed for clinical tests, requiring further testing and validation (Brito et al., 2018). Alternative procedures that can assist in the diagnosis of malabsorption from classic pernicious anemia arising from an autoimmune disease or from long-term atrophy or atrophic gastritis, include a combination of anti-intrinsic factor antibodies, and gastrin and pepsinogen A and C in serum (Green et al., 2017). These tests can all be performed using serologic assays, and if used in combination they can identify most cases of malabsorption from pernicious anemia (Lindgren et al., 1998).22b.2.8 Multiple biomarkers
Defects in the hematopoietic system do not generally occur until the final stage of B12 deficiency. At this stage, the hematological defects are indistinguishable from those of folate deficiency and include megaloblastic anemia, characterized by macro-ovalocytic erythrocytes, abnormal red cell indices, and a low hemoglobin concentration (see Chapter 17, Sections 17.2.7 and 17.4). However, identifying impaired vitamin B12 status in the earlier stages is more challenging, but can be enhanced by using one or more plasma biomarkers of B12 status. No single biochemical biomarker is suitable for diagnosing B12 deficiency in all persons, and the use of several biomarkers is preferred. For a useful summary table comparing the relative strengths and weaknesses of the B12 biomarkers, see Allen et al. (2018). Algorithms based either on a selection of biomarkers measured sequentially or, multiple biomarkers analyzed simultaneously and then combined into a single diagnostic indicator, are both approaches that are used to circumvent the inherent limitations of each individual biomarker. Algorithms based on the selection of sequential assays are used by several laboratories to assess B12 status. For example, initially, serum total B12 or serum holoTC can be measured, and if the concentration is deemed low (i.e., serum B12<148pmol/L or serum holoTC<35pmol/L), then B12 deficiency is diagnosed.
Serum holoTC | Serum total B12 | Plasma MMA | Plasma tHcy | Possible diagnosis |
---|---|---|---|---|
within ref. range | within ref. range | elevated | elevated | Suboptimal B12 status |
decreased | decreased | within ref. range or elevated | elevated | Mild B12 deficiency, on antibiotics |
within ref. range | within ref. range | elevated | within ref. range | Bacterial overgrowth, B12 replete |
very very low | very very low | highly elevated | highly elevated | Pernicious anemia |
within ref. range or decreased | decreased | within ref. range or elevated | within ref. range | Pregnancy, B12 replete |
decreased | very low | elevated | within ref. range or elevated | Pregnancy, B12 deficiency |
within ref. range | within ref. range | within ref. range | elevated to very highly elevated | Mild to severe folate deficiency, B12 replete |
within ref. range or elevated | very low | within ref. range | within ref. range | Haptocorin deficioency |
very very low | within ref. range or decreased or elevated | highly elevated | highly elevated | Transcobalamin deficiency |
within ref. range | within ref. range | very highly elevated | very highly elevated | CblC, D. F, J, disorder |
within ref. range | within ref. range | very highly elevated | within ref. range | CblA, B disorder |
within ref. range | within ref. range | within ref. range | very highly elevated | CblE, G disorder |
within ref. range or decreased | within ref. range or decreased | within ref. range or elevated | very highly elevated | Nitrous oxide abuse |
highly elevated | highly elevated | within ref. range | within ref. range | On Vitamin B12 injections |
very highly elevated | very highly elevated | within ref. range | within ref. range | Chronic myeloid leukemia |
For researchers and clinicians | For epidemiologic purposes | |||
---|---|---|---|---|
Classification | Biological interpretation | Guidelines | Class | Guidelines |
Elevated B12 >1.5 | The pathogenesis of high B12 is not fully understood |
Consider possible causes of high concentrations such as liver disease or current or recent supplementation or treatment | B12 adequacy > −0.5 | No action |
B12 adequacy −0.5 to 1.5 | Expected to accomplish all B12 status–dependent functions | No action advised | ||
Low B12 −1.5 to −0.5 |
Potential subclinical manifestations of B12 deficiency, i.e., absence of hematologic changes, but subclinical neurologic impairment | Consider recommending oral supplements | Transitional B12 status −0.5 to −2.5 | Need fortification |
Possible B12 deficiency < −2.5 |
Potential manifestations of B12 deficiency | Potentially prescribe oral supple- ments, assess again in 3‑6mo | Low B12 status < −2.5 | Need supplement- ation, rechecking, monitoring of status over time |
Probable B12 deficiency < −2.5 | It is possible to observe clinical manifestations of B12 deficiency. Clinical outcomes are needed to confirm potential clinical deficiency |
Consider immediate treatment with i.m.injections, determine cause, consider particularly possible pernicious anemia |